Whereas fission involves the breaking apart of heavy elements such as uranium or plutonium into smaller ones and the release of energy in the process, nuclear fusion involves the “fusing” of light elements, such as hydrogen, into larger ones, such as helium, releasing even greater amounts of energy. Nuclear fusion is the process that powers stars.
Before we go any further, let’s review Einstein’s mass-energy equivalence formula, which is a consequence of special relativity and explains why fusion and fission reactions create so much energy.
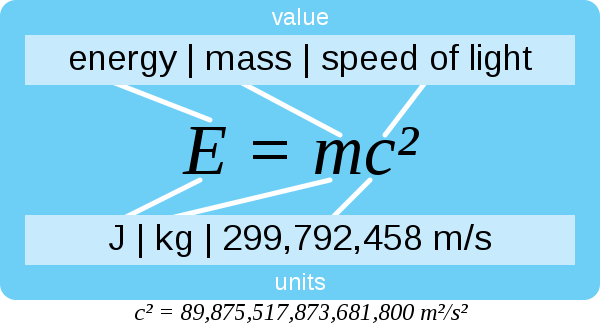
Credit: Wikimedia user JtBarnabas
Einstein’s famous mass-energy equivalence formula says that the amount of energy in an object is equal to its mass times the speed of light (c) squared. The formula says all types of weird things… for example, if you add energy to an object, its mass will increase by a tiny amount even though no matter has been added. Likewise, it says that when mass is lost, as it is in nuclear fission and fusion, tremendous amounts of energy are released. This is because the amount of mass lost is multiplied by the speed of light squared (c2), and as you can see at the bottom of the picture above, c2 is quite a large number!
Nuclear fusion on Earth – both in bombs and in our attempts to make a viable thermonuclear reactor, has involved fusing hydrogen into helium. For the fusion reactors currently being tested, we take two different types of hydrogen atoms, or isotopes: deuterium and tritium. Deuterium, often known as “heavy hydrogen,” is hydrogen with one neutron in addition to the one proton and electron, and tritium, which is very rare naturally but can be synthesized from lithium, has two neutrons, a proton, and an electron. Any atom that has one proton is a hydrogen atom.
For any sort of fusion reaction to occur, the nuclei must be squeezed together. The main obstacle they have to overcome is that the protons are positively charged (neutrons have no charge). Like charges repel, so fusing an atom requires overcoming the repulsive force between the protons in the nucleus.
To overcome this, you need two things: extremely high temperatures and incredibly high pressure. The sun uses the force of gravity to compress and heat the matter in its core, which is 15 million degrees Celsius and is where the fusion takes place. On Earth, we have trouble creating pressures as high as the sun, so we need even higher temperatures – a minimum of 45 million degrees Celsius for Deuterium-Tritium reactions – to fuse elements together. Other fusion reactions, such as Deuterium-Deuterium fusion reactions, require minimum temperatures of 400 million degrees with the pressures we can currently generate.
Thermonuclear Reactors
There are two types of fusion reactors that are currently being explored: magnetic confinement and inertial confinement. Let’s now take a look at how those work.
Magnetic Confinement
A magnetic confinement reactor is a reactor that uses electric and magnetic fields to heat and compress electrified hydrogen gas (hydrogen plasma). The main magnetic confinement reactor that scientists from all over the world are collaborating on is located in France and is called the International Thermonuclear Experimental Reactor (ITER).
The picture above shows a highly simplified schematic of a magnetic confinement chamber. Notice how the main chamber is in the shape of a big donut. This shape is called a toroid, and it happens to be the most efficient shape for confining this plasma. A magnetic confinement chamber in this shape is called a topamak. Toroids have many useful applications in electromagnetics; I know for certain that many of the alternating current transformers I have in my audio amplifiers are in the shape of toroids.
The picture below gives a little more in-depth version of the parts that make up a tokamak. I’ll list them below.
The vacuum vessel: confines the plasma and keeps the reaction chamber in a vacuum
Neutral beam injector (aka: ion cyclotron system): heats plasma by injecting particle beams from the accelerator into the plasma
Magnetic field coils (poloidal, toroidal): magnets that use their magnetic fields to confine the plasma and allow fusion to occur
Transformers/Central solenoid: supply the magnetic field coils with the massive amounts of electricity needed for them to maintain their extremely strong magnetic fields
Cooling equipment (cryostat, cryopump): the magnets generate a lot of heat, so the cryostat and cryopump cool them
Blanket modules: absorb free neutrons and excess heat from the fusion reaction
Diverters: remove excess helium formed from the fusion of deuterium and tritium from the chamber
The fusion reaction becomes initiated when neutral particle beams, electricity, and microwaves from various accelerators heat a mass of hydrogen gas. When this gas is heated to a sufficient temperature, it turns into plasma. Plasma is the same type of substance that stars are made out of and is regarded as a fourth state of matter (regardless of what your elementary science school teacher may have told you, there are more than just three phases of matter!). Power is supplied to the transformers to create a magnetic field (a flowing current of electricity creates a magnetic field around it), and under this extremely strong magnetic field, the plasma is compressed and fusion takes place. Well, at least that’s the idea.
Inertial Confinement
While magnetic confinement works by magnetically compressing the hydrogen ions in close proximity to each other for a given amount of time, inertial confinement works by fuse them so fast that the ions are not able to overcome their inertia and move apart, leading to the name “inertial confinement.”
When I think of inertial confinement reactors, I often get this image of this kid shoplifting a candy bar from a store at night and running away before being surrounded by police pointing a bunch of flashlights at him. Except, in this example, the “kid” is a pea-sized pellet containing deuterium and tritium and the “flashlights” are dozens of incredibly powerful laser beams. The biggest inertial confinement reactor in the world, the National Ignition Facility (NIF) at Lawrence Livermore Laboratory in Livermore, California, contains 192 of these lasers. In the NIF, these lasers are housed within a 10-foot diameter rugby-shaped “target chamber” called a hohlfram. Much like the donut-shaped topomak, I suspect that the geometry of the hohlfram had to do more with efficiency than the engineer’s favorite sport.
The lasers at the NIF will focus 1.8 million joules of energy onto the little pellet of deuterium and tritium, heating it and generating x-rays emanating from pellet. The deuterium and tritium will then turn into plasma as a result of the immense heat and radiation and compress until fusion occurs. Once this fusion occurs, the intense amount of heat and energy released from it will act to sustain fusion. We have not reached this point yet, but we are making progress; back in 2013, we generated a net gain in power produced for the first time in history.
The general mechanism for the generation of electricity for a topamak is the same as any fission or fossil fuel reactor; a reaction produces heat that boils water, creates steam, and drives a steam turbine, creating electricity. So yes, fusion reactors will still have those awesome steam towers.
Benefits of Fusion Power To Fission Power
Abundance of fuel source: Deuterium is very common in the ocean, and tritium can be easily processed from lithium. Our current deuterium/lithium reserves would last us 60 million years, but if we used just deuterium (as we hope to in the future), our fuel reserves would last 150 billion years. To put things in perspective, that’s almost 11 times the age of the universe. Even if we become an incredibly power-hungry civilization and last for five billion more years (until the sun dies), we will have hardly put a ding in these reserves. So for all practical purposes, fusion, particularly deuterium-deuterium fusion, is a renewable source of energy. Uranium, on the other hand, is rare and must be mined.
No long-lived radioactive waste: Fusion reactors produce less radiation than conventional fission reactors, and while waste is produced, it decays on decadal timescales and is approximately as radioactive as coal ash after 100 years. Uranium and plutonium take thousands of years to degrade to safe levels.
No danger of a nuclear meltdown: There’s no danger of a runaway meltdown like there is in a fission reactor; fusion requires incredibly specific conditions to exist, and if these conditions are disrupted due to an external factor like a massive earthquake, the fusion will cease.
More efficient: Nuclear fusion produces much more energy than nuclear fission per unit of fuel.
Is nuclear the fusion the answer for all of our energy problems? Yes and no. It is the “holy grail” of clean energy and something that we should aspire to, but with greenhouse gases accumulating as fast as they are, we need to invest in proven technologies that do not emit carbon dioxide.
Written by Charlie Phillips – charlie.weathertogether.net. Last updated 12/1/2017